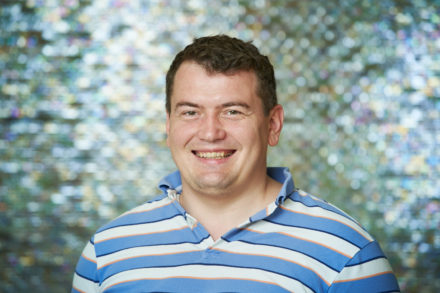
-
Dmitry Kurouski
- Associate Professor, Biochemistry and Biophysics, Biomedical Engineering
- Focus Area: Raman-based plant disease diagnostics, development of nano-infrared spectroscopy (afm-ir) for structural characterization of amyloids and plant waxes, photo- and electrochemical catalysis at nanosclae, forensics
- Office:
- BICH 216
- Email:
- dmitry.kurousky@ag.tamu.edu
- Phone:
- 979-458-3448
Education
- Undergraduate Education
- B.S. Belarussian State University (2008)
- Graduate Education
- Ph.D. SUNY at Albany, State University of New York, 2013 (2013)
- Postdoc. Northwestern University (2013-2015)
Areas of Expertise
- Structural biology
- Optical sensing
- Nanoscale imaging
- Amyloid biology
- Raman spectroscopy
Professional Summary
- Amyloid diseases
The abrupt aggregation of misfolded proteins is linked to the onset and spread of amyloidogenic diseases, including diabetes type 2, systemic amyloidosis, Alzheimer’s (AD) and Parkinson’s diseases (PD). Although the exact cause of these pathological processes is unknown, a growing body of evidence suggests that amyloid diseases are triggered by misfolded or unfolded proteins forming highly toxic oligomers. These transient species exhibit high structural and morphological heterogeneity. Protein oligomers can also propagate into β-sheet rich filaments that braid and coil with other filaments forming amyloid fibrils, supramolecular structures with both flat and twisted morphologies. Microscopic examination of protein deposits formed in the brain of both AD and PD patients revealed the presence of fragments of lipid membranes. These findings demonstrated that lipids bilayers could play an important role in the aggregation of misfolded proteins.
Expanding upon this, we investigate the extent to which (i) lipids can change the aggregation rate of amyloidogenic proteins and (ii) alter the secondary structure and morphology of protein oligomers and fibrils formed in their presence. We utilize state-of-the-art biophysical methods to answer these questions. Using cell cultures, we also (iii) investigate the relationship between the secondary structure of protein aggregates and their toxicity.
Our experimental results suggest that amyloidogenic diseases could be caused by pathological changes in the lipid composition of both plasma and organelle membranes, which, in turn, may trigger protein aggregation that results in the formation of highly toxic oligomers and fibrils.
2. Diagnostics of biotic and abiotic stresses on plants
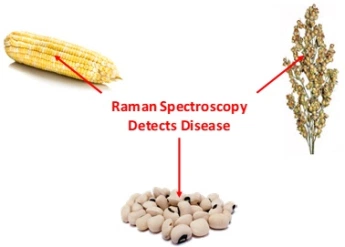
Raman spectroscopy is capable of highly accurate diagnostics of plant diseases and identification of plant varieties. See Trends Analyt. Chem. 118, 43–49 (2019).
As the human population grows from its current size of 7 billion to the projected 9.7 billion in 2050, we will need to produce ~70% more food. These demands can be met by continuous improvement of crop productivity and minimization of losses associated with biotic and abiotic stresses. However, all currently available technologies for detection of plant disease, drought, or nutrient deficiencies are either inefficient or too expensive for farmers and plant breeders. To overcome this problem, we develop the use of Raman spectroscopy for the confirmatory and non-invasive diagnostics of biotic and abiotic stresses in plants. Using Raman spectroscopy, we aim to achieve rapid, label-free, non-invasive and quantitative diagnostics of viral, bacterial, and fungal diseases on a large variety of plant species. We also develop Raman spectroscopy for pre-symptomatic diagnostics of abiotic stresses, including drought and nutrient deficiencies caused by a lack of nitrogen, phosphorus, and potassium. Lastly, we explore the potential of Raman spectroscopy for non-invasive plant phenotyping. Our ultimate goal is to make this Raman-based diagnostic approach broadly available for farmers and plant breeders in the U.S and abroad.
3. Diagnostics of Lyme disease
Lyme disease (LD), one of the most prevalent tick-borne diseases in the United States (US), is caused by Borreliella burgdorferi sensu stricto (Bb). To date, in the US, LD diagnostics is primarily based on validated two-tiered serological testing, which overall exhibits low sensitivity among other drawbacks. We discovered that Raman spectroscopy can be used to detect Bb infection in mice and humans. Currently, we test the robustness and reproducibility of this innovative diagnostic approach on a large number of clinical samples.
4.Entomology – Tick and Locust Identification and Prevention
Ticks and their feces. At the Mexico-U.S. border, with the increase in imports and exports every year, trying to keep out economically and medically dangerous vectors and pathogens becomes more and more troublesome. Current techniques for tick discovery on cattle and other livestock passing the border includes inspecting each animal, one-by-one, all over the body, by hand. However early tick life stages and unfed adults can sometimes be too small to feel. In this laboratory, we’ve shown that conventional Raman spectroscopy can allow for the accurate identification of ticks down to species for ticks such as Ixodes scapularis, Rhipicephalus sanguineus, Amblyomma americana, and many more. What’s more interesting is that this analysis was completed using collected tick frass (feces) from animal hides. We aim to bolster the applications and put this easy-to-use, high-throughput, and non-invasive technology into the hands of the people trying to keep ourselves and our agriculture safe.
Grasshoppers or Locusts? The Central American locust (CAL), Schistocerca piceferons, is a significant threat to U.S. agriculture, especially in southern Texas and Florida where swarms are currently managed just south of the U.S.-Mexico border. This project takes advantage of unique resources and recent advancements in locust genomics and Raman spectrometry-based diagnostics to create and assess innovative tools for identifying and monitoring CAL. Detecting CAL in the U.S. is challenging due to various factors. Firstly, CAL displays density-dependent phase polyphenism, meaning locusts raised under different population densities exhibit distinct morphological and behavioral traits. This variation complicates identification, especially considering the potential for devastating swarms under high-density conditions. Additionally, CAL can hybridize with its non-swarming sister species, the American bird grasshopper (S. americana), naturally found in the southeastern U.S. and northern Mexico. While viable hybrid offspring can be produced, the impact of hybridization on morphological identification and the potential introgression of genetic traits for swarming behavior into the non-swarming species are unknown. Thus, ideal diagnostic tools for the CAL must not only be capable of differentiating closely related species, but also provide information about hybridization.
5. Plasmon chemistry
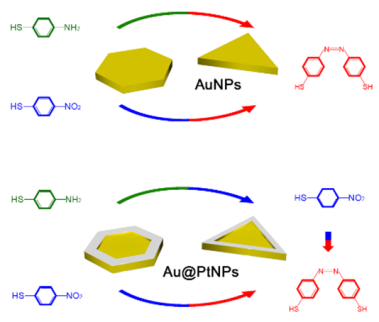
Plasmon driven chemistry at bimetallic nanostructures.
See J. Phys. Chem. C. 124, 12850–12854 (2020).
Plasmonic catalysis is a new emerging direction in organic synthesis. It is based on a unique property of noble metal nanostructures to harvest electromagnetic radiation converting it into hot carriers. These high-energy species can catalyze chemical reactions in molecules located in the vicinity to surfaces of the plasmonic nanostructures. Traditional plasmonic metals such as gold and silver are useful only for a limited number of chemical reactions. Common catalytic metals such as palladium or platinum provide a much broader spectrum of chemical transformations. However, these catalytic metals are not efficient in harvesting electromagnetic radiation. The new paradigm of solid-state catalysis is that coupling of plasmonic and catalytic metals can be used to achieve much higher catalytic efficiency relative to their counterparts. Also, chemical reactions on such bimetallic nanostructures are light-driven, which essentially enables ‘green catalysis’ in organic synthesis. Catalytic efficiency of bimetallic platforms directly depends on their nanoscale structure, which remains poorly understood. Using TERS, we investigate nanoscale catalytic properties of such bimetallic nanostructures. Our goal is to investigate the relationship between nanoscale structural organization of bimetallic nanostructures and their catalytic activity.
6. Forensic analysis of artificial colorants on hair using surface-enhanced Raman spectroscopy (SERS).
Our goal is to develop and deploy surface enhanced Raman spectroscopy (SERS) for confirmatory analysis of colorants directly on hair. We also aim to determine whether SERS can be used to (1) identify whether hair is re-colored and if yes, whether it was re-colored by synthetic permanent, semi-permanent or natural dyes. Finally, we investigate (2) how many dyes can be simultaneously detected on hair and what was the order of their application on hair (which colorant was applied first, which one was used to re-colored it, etc.).
Our recent findings show that SERS could be used to: (i) Identify more than 30 different colorants on hair with nearly 100% accuracy; (ii) Determine whether hair was re-dyed and what is the chemical structure of the underlying colorant. We also investigate the effect of environmental factors, such as UV radiation and temperature on the reliability of SERS-based identification of hair colorants.
Publications
- View publications on Google Scholar